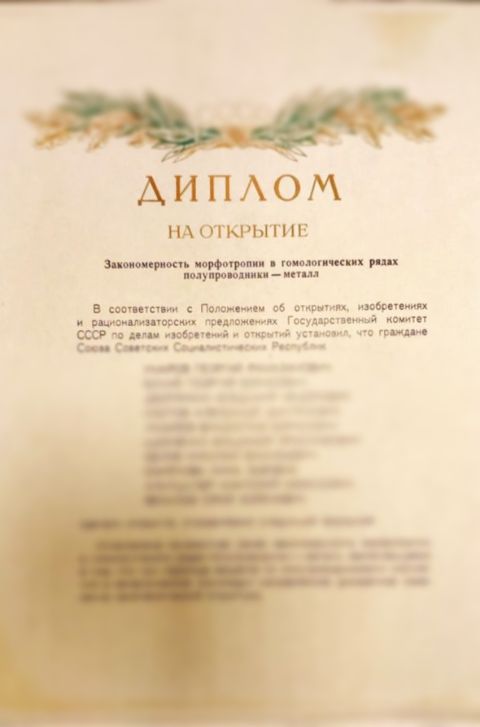
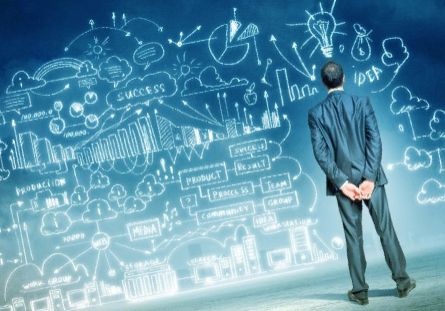
From idea to implementation
High battery performance and efficiency
Energy density
Production costs
forecast
Market overview
The global market for electric vehicle batteries will undergo a radical transformation over the next 12 years. Firstly, it will demonstrate rapid growth—increasing fivefold to $616 billion by 2035. The total capacity of produced batteries will grow even more substantially—almost eightfold, reaching 5.3 terawatt-hours by 2035 compared to the projected 687 gigawatt-hours in 2023.
In other words, it will grow nearly eightfold.
China's position in this market will undergo a significant change—its share in global traction battery production will decrease by half, from the current 75% to 38%. This share will be taken over by new productions in the United States and Europe.
Major consumers of batteries
The main types of batteries today are lead-acid, nickel-based, and lithium-based. Several other types of electric accumulators have been developed and are selectively used in certain industries. They may excel in one specific characteristic as an advantage but fall short compared to lithium, nickel, or acid-based batteries in other parameters. For instance, some may have a record-breaking specific energy capacity but are short-lived, relatively expensive, and inefficient. There is a vast array of experimental or once-tried chemical reaction combinations. Some of them have potential for success in the future, while others are impractical due to identified critical drawbacks (toxicity of components, explosiveness, or high cost).
MARKET EXPECTATIONS
The challenges of growth
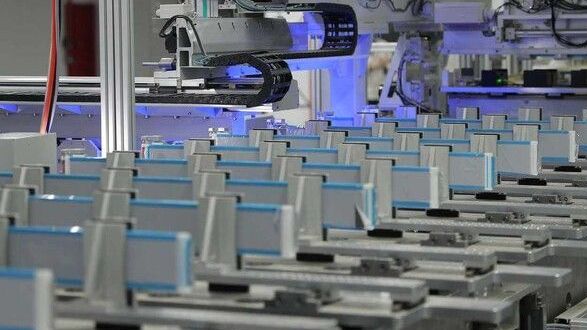
Increase in energy density:
Increasing the amount of energy that can be stored in batteries per unit volume or mass. This will enable the creation of more efficient and long-lasting power sources for electric vehicles, portable devices, and extended autonomous systems.
Fast charging:
Development of technologies that allow quick charging of batteries without risking their life cycle. This will make charging electric devices more convenient and reduce downtime for electric vehicles and appliances.
Durability:
Extending the lifespan of batteries so that they can operate for an extended period without replacement or repair.
Environmental sustainability:
Hybrid and multi-component batteries:
Our group of scientists has managed to find a completely new solution to significantly increase the energy capacity of secondary power sources.
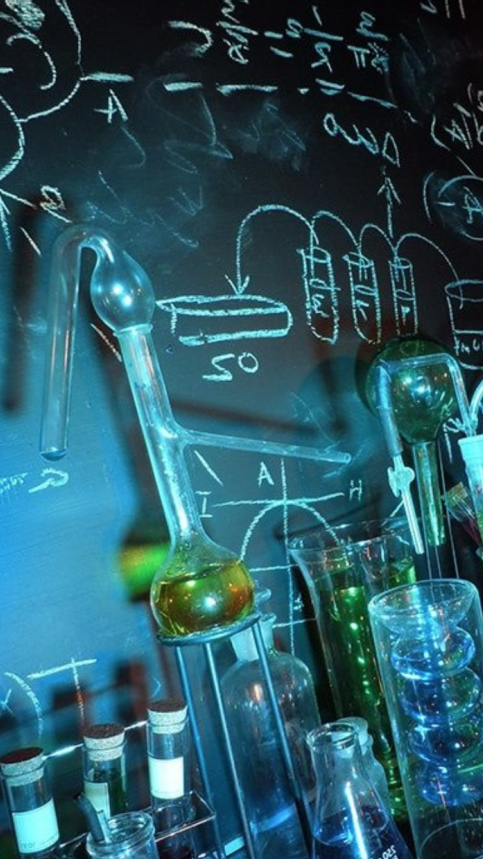
By applying our group's discovered mechanism to autonomous power sources, we have developed a unique technology that allows a significant increase in the capacity of battery cells without a substantial increase in production costs. This is achieved by activating and involving not only valence electrons but also core electrons (i.e., fundamental or deep electrons, the quantity of which in a substance is one to several orders of magnitude higher than valence electrons) in the process of energy storage and release.
The increase in the capacity of batteries through traditionally accepted methods is nearly exhausted today—this involves expanding the interaction surfaces in electrochemical reactions (for example, using graphene). Therefore, achieving a practical increase in specific capacity by approximately 10% is already considered a significant accomplishment.
Suggested Solution
On the other hand, there is a significant reserve that allows for increasing the specific capacity of batteries not by percentages, but by orders of magnitude—these are the core electrons. Mendeleev's table of chemical elements provides data on the quantity of valence and core electrons. For example, the lead atom has 4 valence and 78 core electrons, while the lithium atom has 1 valence and 2 core electrons. Thus, if core electrons are engaged in electrochemical reactions, lead has the potential to increase specific capacity by more than 15 times, whereas lithium can only achieve a threefold increase.
Moreover, lead is much cheaper than lithium, which is expensive and occurs very rarely in nature. Therefore, based on the aforementioned considerations, it is proposed to initiate the implementation of our development with lead-acid batteries. From the perspective of the efficiency of heat engines, the efficiency of lead-acid batteries should be higher, as long as the battery functions as a heat engine. This is due to the higher density of the lead atom's core.
Suggested Solution
The basic schematic of any lead-acid battery includes:
- Cathode
- Anode
- Grid and lead plate
- Electrolyte
- Electronic device and monitoring sensors
The Active Battery will not have any noticeable differences from the standard scheme described above at first glance. But this is only on the surface. In reality, the difference will be colossal.
Firstly, all the materials from which the battery will be made will have a different chemical composition.
Secondly, one or more additional grids will be added.
Thirdly, the chemical composition of the electrolyte will change
Fourthly, the electronic device of the battery will undergo significant changes, essentially transforming into a system for controlling phase transitions. The function of optimizing the battery's operation will be retained but significantly expanded.
Fifthly, several sensors will be added to monitor the processes occurring during phase transformations.
The Active Battery (AB), in addition to the traditional cathode, anode, and electrolyte, also includes:
Active Catalyst (AC): Comprising materials of a new class that activate core electrons.
Electronic Control Unit (ECU): Representing a fundamentally new electronic circuit located inside the battery.
The ECU serves two crucial functions: providing a signal to activate core electrons in the AB and coordinating the parameters of the AB with the external load on the battery.
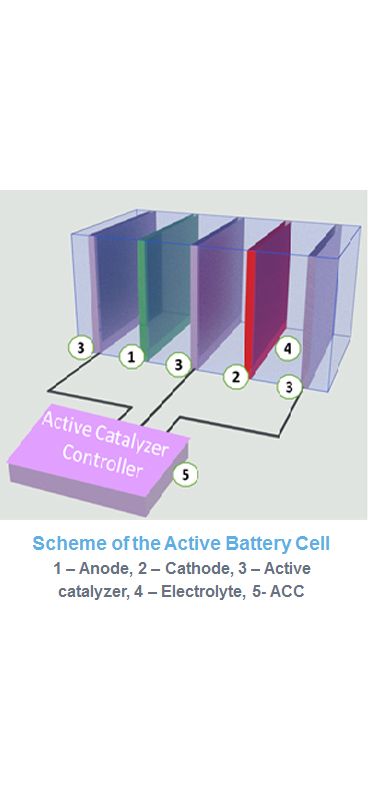
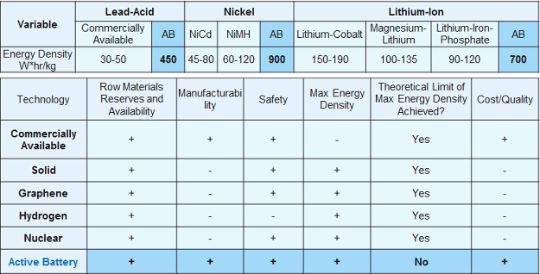
Our project is at an advanced stage of development and implementation. It has a solid scientific foundation and is backed by laboratory results.
OUR TEAM
contact information
CONNECT WITH US
© 2024 All rights reserved.